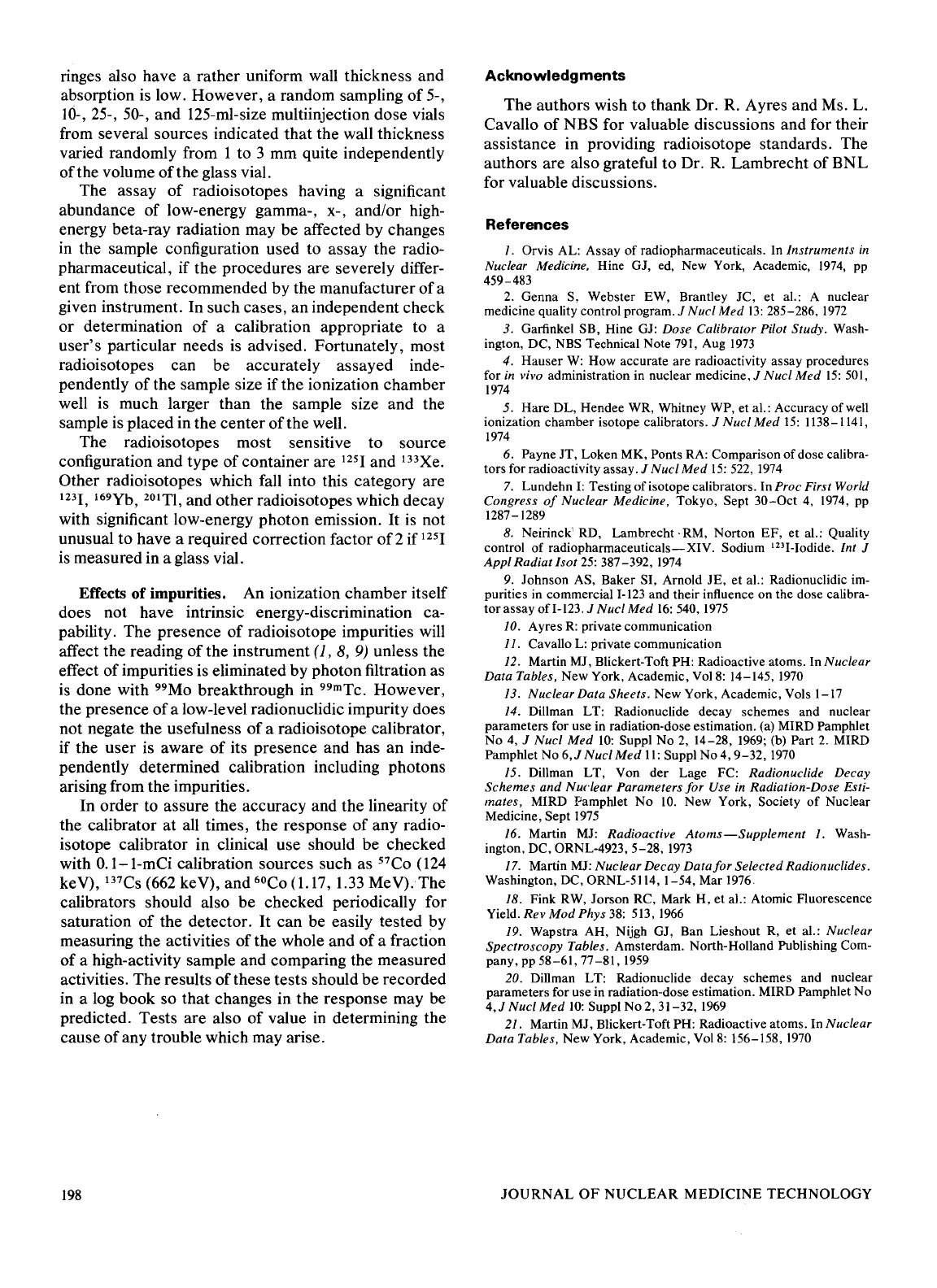
ringes also have a rather uniform wall thickness and
absorption is low. However, a random sampling
of
5-,
Hi-,
25-, 50-, and 125-ml-size multiinjection dose vials
from several sources indicated
that
the wall thickness
varied randomly from I to 3 mm quite independently
of the volume
of
the glass vial.
The assay
of
radioisotopes having a significant
abundance of low-energy gamma-, x-, and/or high-
energy beta-ray radiation may be affected by changes
in
the sample configuration used to assay the radio-
pharmaceutical, if the procedures are severely differ-
ent from those recommended by the manufacturer
of
a
given instrument. In such cases, an independent check
or determination
of
a calibration appropriate to a
user's particular needs
is
advised. Fortunately, most
radioisotopes can be accurately assayed inde-
pendently
of
the sample size if the ionization chamber
well is much larger than the sample size and the
sample is placed in the center
of
the well.
The radioisotopes most sensitive to source
configuration and type
of
container are
125
I and
13
3Xe.
Other radioisotopes which fall into this category are
123
I,
169
Yb,
201
Tl, and other radioisotopes which decay
with significant low-energy photon emission. It
is
not
unusual to have a required correction factor
of2
if
125
I
is measured in a glass vial.
Effects of
impurities. An ionization chamber itself
does not have intrinsic energy-discrimination ca-
pability. The presence
of
radioisotope impurities will
affect the reading
of
the instrument (1,
8,
9)
unless the
effect
of
impurities is eliminated by photon filtration as
is done with
99
Mo breakthrough in
99
mTc.
However,
the presence
of
a low-level radionuclidic impurity does
not negate the usefulness
of
a radioisotope calibrator,
if the user is aware
of
its presence and has an inde-
pendently determined calibration including photons
arising from the impurities.
In order to assure the accuracy and the linearity
of
the calibrator
at
all times, the response
of
any radio-
isotope calibrator in clinical use should be checked
with 0.1-1-mCi calibration sources such as
57
Co
(124
keV),
137
Cs
(662
keV), and
6
°Co (1.17,
1.33
MeV). The
calibrators should also be checked periodically for
saturation
of
the detector.
It
can be easily tested by
measuring the activities of the whole and
of
a fraction
of
a high-activity sample and comparing the measured
activities. The results
of
these tests should be recorded
in a log book so that changes in the response may be
predicted. Tests are also
of
value in determining the
cause
of
any trouble which may arise.
198
Acknowledgments
The authors wish to thank Dr.
R.
Ayres and Ms. L.
Cavallo
of
NBS for valuable discussions and for their
assistance in providing radioisotope standards. The
authors are also grateful to Dr.
R.
Lambrecht of
BNL
for valuable discussions.
References
I. Orvis AL: Assay
of
radiopharmaceuticals. In Instruments
in
Nuclear Medicine, Hine GJ, ed,
New
York, Academic,
1974,
pp
459-483
2.
Genna S, Webster EW, Brantley JC,
et
al.: A nuclear
medicine quality control program.
1
Nucl
Med
13:
285-286,
1972
3. Garfinkel SB, Hine GJ: Dose Calibrator Pilot Study. Wash-
ington, DC,
NBS
Technical Note
791,
Aug
1973
4.
Hauser
W:
How accurate are radioactivity assay procedures
for
in
vivo administration in nuclear medicine, 1
Nucl
Med
15:
501,
1974
5.
Hare DL, Hendee WR, Whitney WP, et al.: Accuracy
of
well
ionization chamber isotope calibrators.
1
Nuc/
Med
15:
1138-1141,
1974
6. Payne JT, Loken MK, Ponts
RA:
Comparison
of
dose calibra-
tors for radioactivity assay.
1
Nucl
Med
15:
522,
1974
7.
Lundehn
I:
Testing
of
isotope calibrators. In Proc First World
Congress
of
Nuclear Medicine, Tokyo, Sept
30-0ct
4,
1974,
pp
1287-1289
8.
Neirinck' RD, Lambrecht· RM, Norton
EF,
et
al.: Quality
control
of
radiopharmaceuticals-XIV.
Sodium
123
I-Iodide.
lnt
1
Appl
Radiat
/sot
25:
387-392,
1974
9.
Johnson AS, Baker SI, Arnold JE,
et
al.: Radionuclidic
im-
purities in commercial
I-123
and their influence on the dose calibra-
torassayofl-123.1
NuclMed
16:540,1975
10. Ayres
R:
private communication
11.
Cavallo
L:
private communication
12.
Martin MJ, Blickert-Toft PH: Radioactive atoms. In Nuclear
Data Tables,
New York, Academic, Vol8: 14-145,
1970
13.
Nuclear Data Sheets. New York, Academic, Vols 1-17
14.
Dillman LT: Radionuclide decay schemes and nuclear
parameters for use in radiation-dose estimation. (a) MIRD Pamphlet
No 4,
1
Nuc/
Med
10:
Suppl No
2,
14-28,
1969;
(b) Part
2.
MIRD
Pamphlet No
6,1
Nuc/
MedII:
Suppl No 4,
9-32,
1970
15.
Dillman
LT,
Von der Lage FC: Radionuclide Decay
Schemes
and
Nuclear Parameters
for
Use
in
Radiation-Dose Esti-
mates,
MIRD Pamphlet
No
10.
New York, Society
of
Nuclear
Medicine,
Sept
1975
16.
Martin MJ: Radioactive
Atoms-Supplement
1.
Wash-
ington, DC, ORNL-4923,
5-28,
1973
17.
Martin MJ: Nuclear Decay Data
for
Selected Radionuc/ides.
Washington, DC, ORNL-5114,
1-54,
Mar
1976
18. Fink RW, Jorson RC, Mark
H,
et
al.: Atomic Fluorescence
Yield.
Rev
Mod
Phys
38:
513,
1966
19.
Wapstra AH, Nijgh GJ, Ban Lieshout
R,
et
al.: Nuclear
Spectroscopy Tables.
Amsterdam. North-Holland Publishing Com-
pany, pp
58-61,
77-81,
1959
20. Dillman LT: Radionuclide decay schemes and nuclear
parameters for use in radiation-dose estimation. MIRD Pamphlet No
4,1
Nuc/
Med
10:
Suppl No 2, 31-32, 1%9
21. Martin MJ, Blickert-Toft PH: Radioactive atoms. In Nuclear
Data Tables,
New York, Academic, Vol8: 156-158,
1970
JOURNAL
OF
NUCLEAR MEDICINE TECHNOLOGY